Comply with MIL standards for quality control of military equipment
When choosing what sections of the MIL standards you wish to test your equipment up against, there are a number of aspects concerning immunity and EMC that you need to consider.
When equipment for military use needs to be quality controlled, MIL standards are used that specify the minimum requirements for electrical and mechanical components and devices. The system, which was developed by the USA’s military, is now used as a quality reference all over the world.
For EMC, the MIL standard over all others has, since the 1960s, been 461. The latest version is MIL-STD-461G. When this standard is used, it almost always forewarns some very strict requirements. On the other hand, it is widespread and recognised over most of the world.
Be careful of the scope – it’s huge!
Never make promises to comply with “all of MIL-STD-461”. Without specifying any limitations, this would mean that you would have to comply with both EMP tests and tests for lightning-induced transients and HF radiation at 200 V/m. On the emission side as well, the requirements can be hard to comply with. In general, the equipment class “Ground, Army” can be used, assuming that the customer has not already specified his demands. The relevant tests can be seen in Figure 1, where Ground, Army has 8 x ‘A’, which stands for Applicable Tests.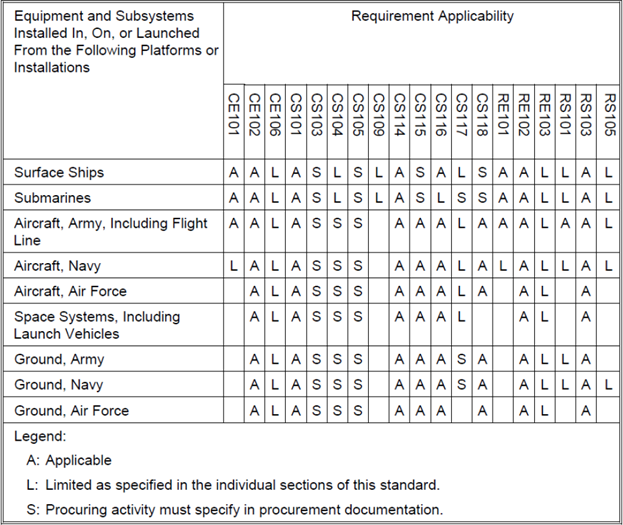
Revision G with numerous approximations
MIL-STD-461G is of US origin and is published by the US Department of Defence. It has thus no relation to EU standards or international IEC standards. MIL-STD-461 contains about 20 test parameters, of which 10-12 are relevant for the majority of the products.The standard is very systematic, with set terms for the different test parameters. All emission test parameters have ‘E’ as the last letter. For example: RE – Radiated Emission for all radiated emission tests. Likewise, immunity parameters are referred to with an ‘S’ for Susceptibility, which is the standard’s term for ‘vulnerability’. Susceptibility is the inverse expression for immunity. For example: Conducted Susceptibility for conducted immunity tests. In addition, the tests have 3-digit serial numbers.
In connection with the publication of version G, the scope of the test has been slightly expanded, so that for example the ESD test CS118 (test with electrostatic discharges) which corresponds completely to the civilian ESD standard IEC 61000-4-2, has been added. In line with the system used in the MIL standard, one special feature has been added however: A mandatory “check” of the test setting before you carry out the test on your product. You must verify that there is actually voltage present at the outlet of the ESD simulator prior to testing, just as you must verify for all the other tests that the test signal is correctly adjusted before completing a test. All emission measurements must contain a set of data, where a known signal is applied to a measuring network, current probe or antenna to verify that it measures as expected. This is built-in quality assurance and thus good laboratory practice.
The MIL standard has also been expanded with a demanding test taken from the aviation world: test CS117. This describes a test with more than five different transients and must be used to verify electronics that shall be used with the risk of induced disturbances from lightning strikes in the area (indirect lightning strikes).
Immunity across the entire frequency area – no exceptions!
The biggest challenge for electronics manufacturers that meet MIL-STD-461 for the first time is the large frequency range. For some types of equipment, the requirements start at 30 Hz, while for HF radiation it ends at 18 or 40 GHz.The large frequency range means that the operational range for EMI filters on power supplies and signal connections must be expanded in relation to ‘the usual’ CE marking requirements. This also means that for example the switch frequency in a power supply cannot be placed outside of the requirement’s frequency area. Test CE102 is very similar to the civilian measurement of Conducted Emissions in relation to CISPR 16-2-3. The same impedance procedure is even used to measure the impedance (50 Ω 50 μ LISN). The requirements start however at 10 kHz, and this can require the design of an extra filter section on the mains/power filter.
The BCI concept – Bulk Current Injection
Tests with conducted signals (CS tests) take place in the frequency range 30 Hz to 200 MHz. Test CS114 is performed on all cables. 200 MHz is so high a frequency that filter components and shielded cables must be well constructed in order to work. And the large frequency range means that there is a very high likelihood of unwanted coupling at the most vulnerable frequency in the system or that frequency where the filters and shields perform at their poorest.The MIL standard uses the BCI probe for immunity testing. Physically, this is a magnetic core with a conductor which the test signal is connected to. The magnetic core can be opened and fixed around a cable. The signal injection is made magnetically, so you do not need to connect the switching network or similar to the test object. A BCI probe can thus be used on all types of cables.
The term ‘bulk’ refers to the fact that the BCI probe connects to all wires in a cable at the same time. Whether a current is actually generated in all of the wires depends on the impedance which each wire ends in (at each end of the cable). So, it makes no sense to carry out BCI coupling to a cable with a blind end. You have to connect cables to peripheral equipment or a simulation box that corresponds to a realistic impedance. The BCI coupling is a Common Mode (CM) coupling. No differential test signals are introduced, so it therefore models a situation where an HF noise signal is transferred from another noisy cable that is led parallel in a cable box, for example. Common Mode signals (induced CM currents) can also occur if a cable captures radio waves through the air.
The test currents in test CS114 can be up to 300 mA. A current as powerful as this can destroy components in an interface circuit if filter components are not used that can handle and reduce the current.
The powerful electromagnetic fields
The most challenging immunity test for MIL clients is RS103: HF radiation in the frequency area 2 MHz to 40 GHz. Certain test classes can manage with the frequency area 30 MHz to 18 GHz. Under all circumstances, the broad frequency range is a challenge. Cable shields already often start to lose their effect already below 1 GHz. Even good cabinets start to leak at frequencies above 1 GHz, and filters on circuit boards must be carefully completed and with ground plane layer in order to be effective above 1 GHz. Once again, the broad frequency range means that at some point or another, the test frequency will meet precisely that frequency where filter damping is minimal and the circuit is sensitive. This is the exact equivalent of a worst-case scenario, where a radio frequency or perhaps a broadband noise signal from a motor controller occurs in the use environment for a product.A field strength of 200 V/m is the strongest test class in the standard. This is used for equipment that is installed on aircrafts or on open decks of a ship. The ‘lower’ test classes are at 50 V/m and down to 10 V/m, which is the weakest. 10 V/m is the test class which civilian standards use for industrial equipment.
In order to be able to generate field strengths of 200 V/m in laboratory settings, you need to use amplifiers that are in KiloWatt scale. In the frequency range of 30-100 MHz it can be especially hard, as you need a large antenna that you must also be able to position 1 m from the test subject. In the range above 1 GHz, power amplifiers are an expensive facility. The standard does allow however the use of a reverberation chamber, which with its resonance structures (see Figure 2) can create a constructive amplification of the wave patterns, so you can achieve the 200 V/m or more with reasonable amplification. Civilian standards currently describe radiation testing up to 6 GHz. Commercial HF amplifiers with power transistors have thus become widespread.
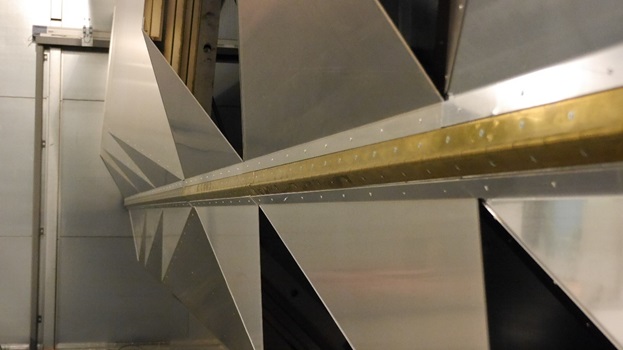
TWT – travelling wave amplifiers
If you need to go above 6 GHz, the amplifiers often have a built-in TWT (Travelling Wave Tube). The TWT can be described as an experimental, physical structure installed in an amplifier cabinet. The principle is shown in Figure 3 and consists of transferring the energy from a powerful electron beam to the same frequency as the HF signal, which is coupled to the TWT’s input.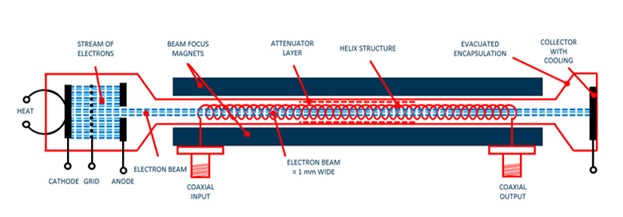
The electrons are formed by heating up the cathode with a filament. The anode and the grid release the electrons to form the electron beam that passes through the tube. The long helix structure is used to create a slowly moving wave from left to right on the figure. The HF signal spreads across the helix surface at about the speed of light, but due to the incline on the helix structure, the wave structure in the tube is somewhat slower than the speed of light. Along the helix there is an interaction between the signal that is fed into the input and the electron beam. The local electric field strengths close to each helix conductor accelerate or brake the electrons in the beam, and the electrons in the beam gather in ‘clumps’, which match the frequency of the input signal. The interaction is mutual, so the electron beam induces a signal in the helix structure and the amplification in the passage through the tube is great (perhaps 100 times). At the end of the tube, part of the electron beam’s energy is transferred to the helix structure and removed from the output connector. The remaining energy is delivered upon the electron beam’s collision with the collector electrode. It requires a great deal of experience to be able to build and produce a TWT, and only a handful of manufacturers in the world (such as Teledyne, CPI and L3 EED) produce tubes and amplifiers. TWT amplifiers with the highest band widths can handle a frequency area of 2 decades, e.g. 2-8 GHz. A very small production volume keeps the prices high, and together with several powerful high voltage power supplies, helps to make the amplifiers extremely costly.
Noise and distortion
Besides the price and complexity, the amplifiers are difficult because they generate a pretty coarse signal, which combined with the desired signal includes some noise and harmonic overtones. An example of this is shown in Figure 4, where A shows the input and output signal on a 2-8 GHz TWT amplifier of 250 W. With the use of the output filter, input- and output signals remain reasonably the same, with a satisfactory signal. Without the filter (B) the signal becomes more distorted. There are differences in the positive and negative half period, which shows the content of equal harmonic overtones in the signal. It can impact EMC test results if the distortion becomes so powerful that the amplifier is almost testing several frequencies at the same time.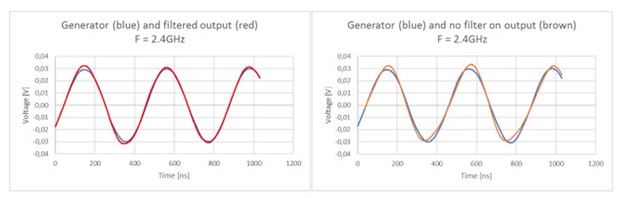
Abbreviations
BCI: Bulk Current InjectionEN: European Norm
EU: European Union
EUROCAE: European Organisation for Civil Aviation Equipment
IEC: International Electrotechnical Commission
ISO: International Standardisation Organisation
LISN: Line Impedance Stabilization Network
RTCA: Radio Technical Commission for Aeronautics